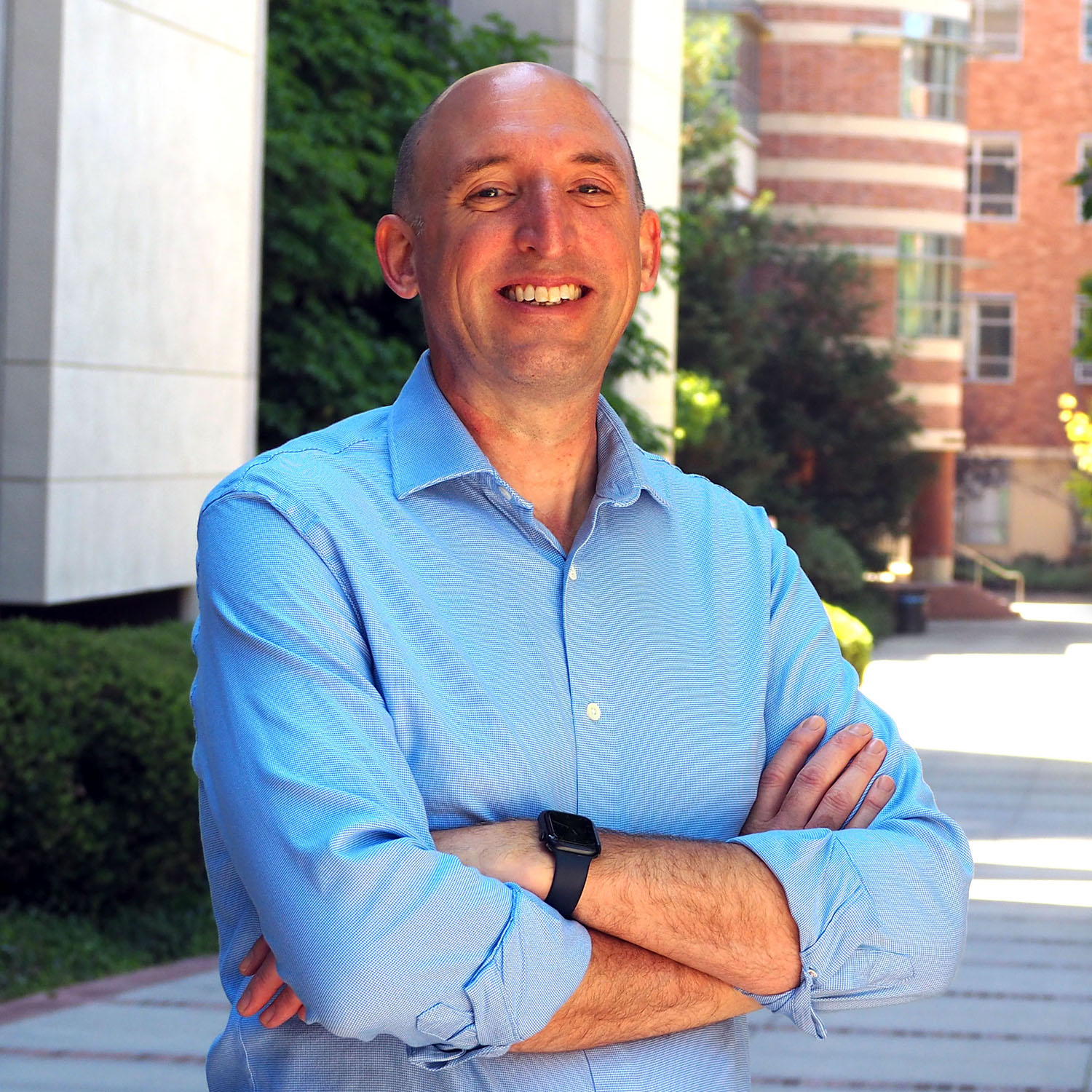
Troy Carter
Professor
Department of Physics and Astronomy
Director
Plasma Science and Technology Institute
4-909 Physics & Astronomy Building (PAB)
Los Angeles, CA 90095-1547
310-825-4770
tcarter@physics.ucla.edu
Troy Carter is a Professor of Physics at the University of California, Los Angeles. Prof. Carter is the Director of the Basic Plasma Science Facility (BaPSF), a national user facility for plasma science supported by DOE and NSF. He is also the Director of the Plasma Science and Technology Institute (PSTI), an organized research unit at UCLA. His research into waves, instabilities, turbulence and transport in magnetically confined plasmas is motivated by the desire to understand processes in space and astrophysical plasmas as well as by the need to develop carbon-free electricity generation via nuclear fusion. Prof. Carter led the DOE FESAC Long Range Planning process that resulted in the 2021 report “Powering the Future: Fusion and Plasmas.” He is a Fellow of the APS and is a recipient of the APS DPP John Dawson Excellence in Plasma Physics Research Award and of the Fusion Power Associates Leadership Award. Prof. Carter received BS degrees in Physics and Nuclear Engineering from North Carolina State University in 1995 and a PhD in Astrophysical Sciences from Princeton University in 2001.
To learn more about recent research and publications, visit my Google Scholar page.
To learn about current projects and group members, visit:
Former graduate students
- Gurleen Bal (2023, Asst. Teaching Professor (LPSOE), UCSD)
Thesis: RF Sheath Mitigation and RF Wave Coupling Studies for Optimal ICRF Heating - Kamil Sklodowski (2023, scientist, ASML)
Thesis: Laboratory Experiments on Arched Magnetized Plasmas - Shawn Tang (2021, Staff Scientist, General Atomics)
Thesis: Controlled Stabilization of Alfven Eigenmodes in DIII-D and Validation of Theory and Simulations - Giovanni Rossi (2021, Entreprenuer, Actual Education)
Thesis: Electromagnetic Turbulence and Transport in Increased Beta LAPD Plasmas -
Jeffrey Robertson (2020, Senior Software Engineer, Impira)
Thesis: Shear Alfven Waves in Two-Ion Plasmas -
Tom Neiser (2019, Research Scientist,General Atomics)
Thesis: Gyrokinetic simulations of turbulence in the near-edge of fusion plasmas -
Lazlo Bardoczi (2017, Asst. Professor, UC Irvine)
Thesis: Multi-Field/-Scale Interaction of Neoclassical Tearing Modes with Turbulence and Impact on Plasma Confinement - Brett Friedman (2013, Research Scientist, LLNL)
Thesis: Simulation Analysis of Zero Mean Flow Edge Turbulence in LAPD - David Schaffner (2013, Assoc. Professor & Dept. Chair, Bryn Mawr College)
Thesis: Study of Flow, Turbulence and Transport on the Large Plasma Device -
Jie Zhang (2013, Senior Manager, LAM Research)
Thesis: Study of internal magnetic field via polarimetry in fusion plasmas -
Jon Hillesheim (2012, Scientist, Commonwealth Fusion Systems)
Thesis: Studies of turbulence and flows in the DIII-D tokamak - David Auerbach (2011, Machine Learning Engineer, Cash App)
Thesis: Resonant Drive and Nonlinear Suppression of Gradient-Driven Instabilities via Interaction with Shear Alfven Waves - Travis Yates (2010, NRG Energy)
Thesis: Laser-Based Measurements of Magnetic Fluctuation-Induced Particle Transport and Nonlinear Mode Interactions in the Madison Symmetric Torus Reversed-Field Pinch - Warren Lybarger (2009, Aerospace Corporation)
Thesis: Enabling Coherent Control of Trapped-ions with Economical Multi-laser Frequency Stabilization Technology - David Pace (2008, Deputy Director, DIII-D, General Atomics)
Thesis: Spontaneous thermal waves and exponential spectra associated with a filamentary pressure structure in a magnetized plasma -
Anne White (2008, Professor and Dept. Head, MIT)
Thesis: Experimental study of electron temperature fluctuations in the DIII-D tokamak - Brian Brugman (2007, Portfolio Manager, Engineers Gate)
Thesis: An Investigation of the Nonlinear Interaction of Alfven Waves
Former postdocs
Pavel Popovich (Limat Capital, Zurich), Brett Friedman (LLNL), Seth Dorfman (SSI).